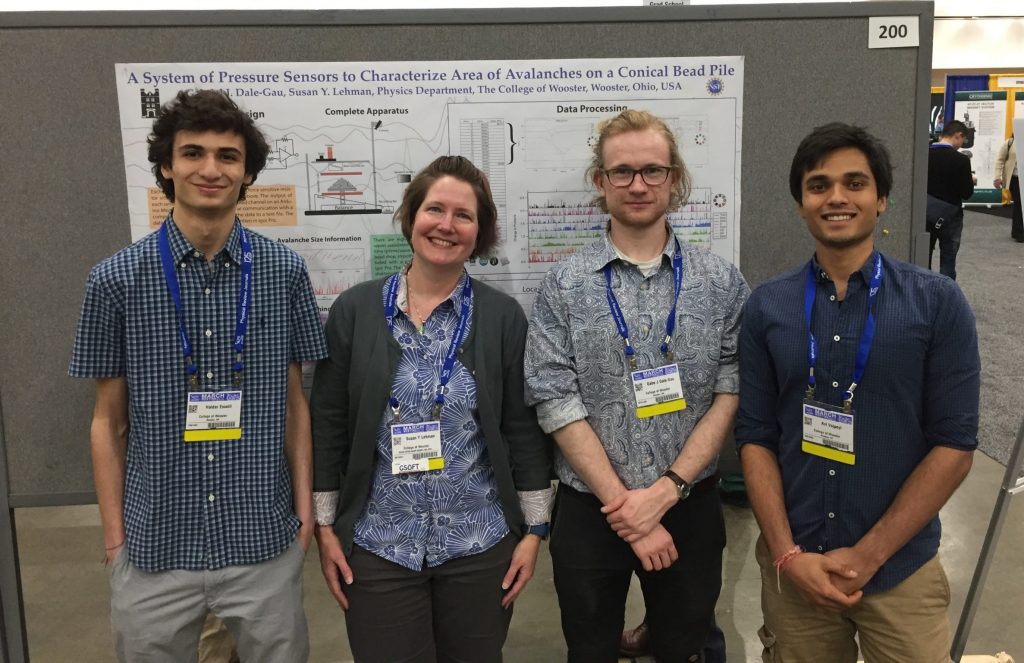
Condensed Matter: All Sorts
Current Experiments
Avalanches on a Conical Beadpile
A granular system behaves in some ways like a liquid with an ability to flow and in some ways like a solid with a stable fixed structure if undisturbed. As a result, the behavior of a granular system such as a beadpile with beads constantly added to the top is complex and fascinating. Before adding a bead, we cannot tell whether the bead will stay on the pile, fall off the pile, cause an avalanche of a few beads, or cause a catastrophically large avalanche. Avalanches are relatively infrequent — most often the bead stays on, building the pile. Small avalanches (measured by the number of beads involved) are much more common than large avalanches, and in fact the probability P of an avalanche occurring depends on its size s as a power law according to

where the exponent τ is near 1.5 in our experimental work.
We have been using a scaling analysis to provide a more complete description of the dynamics of the beadpile system than an analysis only of the critical exponent τ. A preprint of an article summarizing the scaling analysis and our recent results is available on the arXiv, and was published in final version by Granular Matter.
A gif of a sample avalanche on the beadpile. The conical pile geometry makes it difficult to image the full pile. The camera is located about a meter above the pile to minimize visual distortion between beads at the top of the pile and beads at the bottom. There is a slight distortion remaining, however; the camera is not directly above the apex so the pile is just slightly skewed from that perspective.
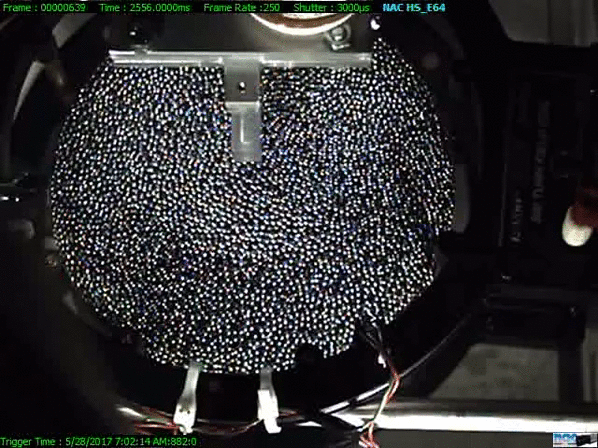
The beadpile has a long history at the College of Wooster under the guidance of Dr Don Jacobs; I began collaborating on this work around 2009, and took over entirely when Dr. J retired in 2010. We are currently collaborating with Dr. Karin Dahmen of the University of Illinois, Urbana-Champaign to investigate the effect of cohesion both in our experimental beadpile and Dr. Dahmen’s theoretical framework. This collaborative work was funded by the National Science Foundation grant CBET-1336116. As part of this grant, we added video tracking to the system in order to provide a fuller perspective on the dynamics of individual avalanches. We are currently working on ways to process and analyze the video results to better understand avalanche behavior at different scales. Students who have worked on the project most recently include Haidar Esseili (Summer Research 2017), Gabe Dale-Gau (Summer 2017 and Senior IS 2018), Kyle McNickle (Senior IS 2018), Katie Shideler (Summer 2018), Montana Ferita (Summer 2018), David Morrow (Senior IS 2019), Bennett Anderson (Summer 2019 and continuing to March 2021), Emmanuel Ogundipe (Summer 2019), Adam Deeley (Senior IS 2020), Melita Wiles (Summer 2020 continuing to March 2021), and Henry Whyte (Senior IS 2021).
Katie receiving her poster award from the March Meeting in Boston. Justine explaining her work in Baltimore. Gabe using multi-media to explain his Senior IS at the IS Symposium.

Scanning Tunneling Microscopy and BEEM
We have a Dimension 3100 scanning probe microscope from digital instruments (now Bruker), which can be used either as an atomic force microscope (AFM) or as a scanning tunneling microscope (STM). We have modified the STM mode to enable a technique known as ballistic electron emission microscopy (BEEM). Scanning tunneling microscopy measures the total current that tunnels between the tip and sample and uses this current to provide an image of the sample. The BEEM technique requires a different type of sample and uses two sample contacts to separate this single current into two. The sample has to have a thin metal overlayer, and most of the tunneling electrons stay one within this layer. A tiny fraction of the injected electrons travel without collisions through the thin metal layer and have enough energy to overcome the energy barrier that has formed between the metal layer and the semiconductor beneath it. This current of the energetic electrons is called the BEEM current. By changing the energy of the injected electrons, we can see at what energy the BEEM current becomes non-zero, and so then we know the electrons had the right energy to overcome the barrier height. In sum, BEEM measures the electronic properties of buried interfaces with high lateral resolution. The delicate and challenging part is that the BEEM current is typically on the order of a few picoamps, so we need excellent electronics to be able to reliably measure this tiny, noisy current.
Nathan Johnson (Senior IS 2016) used the BEEM to study GaN nanowires, though this project has been tricky at best! Students who worked on the project to originally convert the STM to BEEM were Austin Carter (Senior IS 2005), Kathy McCreary (Senior IS 2006), Danny Shai (REU Senior Researcher 2007), and Richard Sampson (REU 2008).
Previous Experiments
Swelling of Sol-gel Derived Organosilica
Osorb ® is a sol-gel derived organosilica that instantaneously swells up to four times in volume with organic liquids. The nanoporous glass-like material is hydrophobic and does not swell in water but absorbs non-polar organic solutes from aqueous solution. When the material swells due to absorption of organic solutes, substantial mechanical force is generated. We have investigated the force exerted by placing a powdered sample in a cylinder with a freely movable piston. As solvent percolates into the cylinder from below, the exerted force is measured by a load cell. The piston is then gradually moved upward to allow the material to expand.
Osorb ® was discovered by Dr. Paul Edmiston of the College of Wooster Department of Chemistry. Our work on Osorb ® in the Physics Department began with Lily Christman (REU 2010) and continued with a number of students, most recently Evan Hagedorn, a chemistry & physics double major (Senior IS 2015). Dr. Paul Bonvallet in the Chemistry Department is continuing this work from the chemist’s perspective. For more information on Osorb ®, please visit ABSMaterials. This work with Dr. Bonvallet and Dr. Edmiston with a theoretical framework developed by my physics colleague Dr. John Lindner and the work of many students was published in the spring of 2020 at AIP Advances.
Atomic Force Microscopy of nanoscale semiconductors
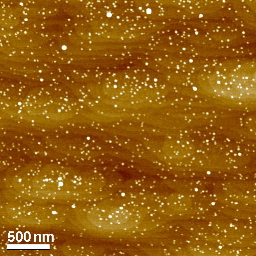
In this atomic force microscope image, the dots appear as bright points. These quantum dots are pyramidal islands of Indium Arsenide (InAs) approximately 5 nanometers tall. The dots are grown on a substrate of Gallium Arsenide (GaAs). The scalloped layers visible across the background are actually steps from one atomic layer to the next in the GaAs crystal.
We are using the scanning probe microscope to investigate a variety of nanostructures, including InAs and InGaAs quantum dots. Quantum dots are structures which are so small (generally < 40 nm) that the free electrons are confined in all three dimensions. The dots are a physical realization of the standard quantum “particle-in-a-box” problem and are sometimes called artificial atoms.
Students who have worked on this project include Annie Erbsen (REU 2004), Stephen Poprocki (REU 2004), and Kelly Patton (REU 2005). This work was in collaboration with Dr. Alexana Roshko at the National Institute of Standards and Technology in Boulder, and resulted in a 2005 publication in the Journal of Vacuum Science and Technology:B, a 2009 publication also in the Journal of Vacuum Science and Technology:B, and another 2009 publication in the Journal of Crystal Growth.
Cavity Ring-down
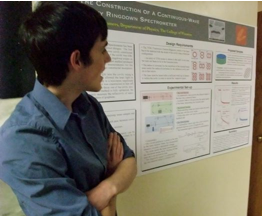
Cavity ring-down is an optical technique which is extremely sensitive to any sources of optical loss within the measurement cavity. We have modified the cavity so that we can easily switch between an end-injected and center-injected geometries. The cavity was mainly used to study semiconductor mirrors known as DBRs. This project is currently in deep hibernation–so far I have not found a good sample system for us to investigate.Students who have worked on this project include Dan Utley (Senior IS 2005), Danny Tremblay (REU 2005), Ian Steward (REU 2007), Henry Timmers (Senior IS 2009), and Heather Moore (Senior IS 2010).
Mpemba Effect
Whether hot water sometimes freezes faster than cold water has been greatly debated. This phenomenon is known as the Mpemba Effect. Joe Thomas began our research in this area with a Junior IS self-designed project; he found some interesting results so we continued the work for his Senior IS (2008). Ingrid Thvedt (REU 2008) continued the project that summer. Martha Roseberry (Senior IS 2009) made some significant improvements to the consistency of our set-up, adding multiple thermistors to monitor the temperature during freezing. Erin Ford (REU 2009) continued data collection with Martha’s modifications. This project is also in hibernation.